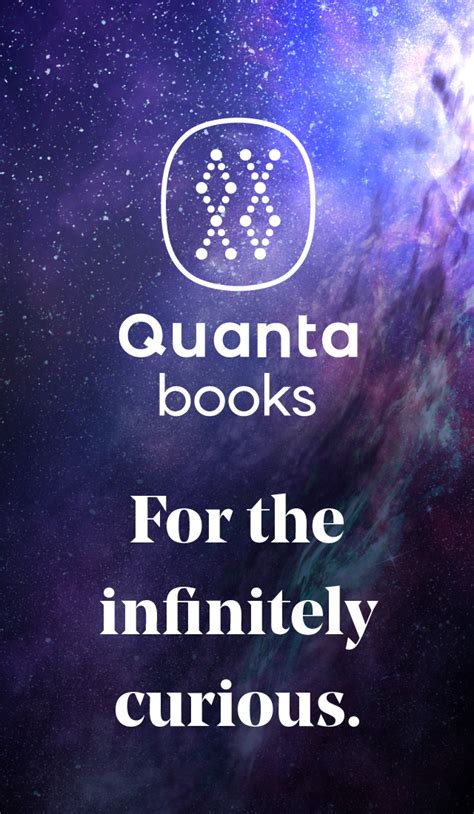
The prevailing understanding of gravity, a force long described by Einstein’s theory of general relativity, may be fundamentally incomplete, prompting physicists to explore alternative models that could reshape our comprehension of the cosmos, dark matter, and dark energy. Researchers are challenging the assumption that gravity is solely a geometric property of spacetime, suggesting that other forces or particles might play a crucial role in mediating its effects, particularly at cosmological scales.
The existing model of gravity, as described by Einstein’s general relativity, has stood as a cornerstone of modern physics for over a century. It explains gravity as the curvature of spacetime caused by mass and energy, accurately predicting phenomena like gravitational waves and the bending of light around massive objects. However, it falters when confronted with the mysteries of dark matter and dark energy, which together constitute approximately 95% of the universe’s total mass-energy content. These enigmatic entities, inferred from their gravitational effects but never directly observed, have led some physicists to question whether our understanding of gravity itself is flawed.
“We might be completely wrong about gravity,” stated the original Yahoo News article, encapsulating the bold re-evaluation underway in the scientific community. This isn’t to say that general relativity is entirely incorrect; it remains remarkably accurate within the Solar System and in other strong gravitational fields. Instead, the issue lies in its inability to account for the universe’s accelerating expansion and the observed dynamics of galaxies without invoking dark matter and dark energy – placeholders that, despite decades of research, remain elusive.
Modified Newtonian Dynamics (MOND) is one such alternative theory gaining traction. MOND proposes that at very low accelerations, such as those experienced by stars in the outer reaches of galaxies, gravity deviates from Newtonian predictions. This modification could explain the observed flat rotation curves of galaxies – where stars orbit at roughly the same speed regardless of their distance from the galactic center – without the need for dark matter. While MOND successfully predicts certain galactic phenomena, it struggles to provide a comprehensive cosmological framework and has difficulties explaining the behavior of galaxy clusters.
Another line of inquiry involves exploring the possibility that gravity is mediated by particles, similar to how the electromagnetic force is mediated by photons. Some theories posit the existence of hypothetical particles called “gravitons” that carry the gravitational force. If gravitons exist and interact with other particles in unexpected ways, this could lead to modifications of gravity at large scales. Furthermore, these gravitons could interact with hypothetical dark matter particles, potentially explaining some of the observed gravitational effects attributed to dark matter.
Beyond MOND and graviton-based models, other theoretical approaches involve modifying Einstein’s field equations or introducing new fields that interact with gravity. Some of these theories suggest that dark energy isn’t a separate entity but rather a manifestation of modified gravity at cosmological distances. These modifications aim to reconcile general relativity with the observed accelerated expansion of the universe without resorting to the exotic properties of dark energy.
Experiments are crucial to validating or refuting these alternative theories. Astronomers are conducting precise measurements of galaxy rotation curves, gravitational lensing, and the cosmic microwave background to search for deviations from general relativity’s predictions. Space-based missions, such as the European Space Agency’s Euclid telescope, are designed to map the distribution of galaxies and dark matter with unprecedented accuracy, providing a wealth of data to test different gravity models.
The search for a more complete theory of gravity has profound implications for our understanding of the universe. If successful, it could not only solve the mysteries of dark matter and dark energy but also revolutionize our understanding of cosmology, astrophysics, and fundamental physics. The potential rewrite of gravity promises a deeper, more nuanced view of the universe’s fundamental forces and the very fabric of spacetime.
In-Depth Analysis:
The current standard model of cosmology, known as Lambda-CDM, relies heavily on the existence of cold dark matter (CDM) and a cosmological constant (Lambda) to explain the observed structure and expansion of the universe. While Lambda-CDM provides a good fit to many cosmological observations, it faces several challenges, including the aforementioned lack of direct detection of dark matter particles and the fine-tuning problem associated with the cosmological constant. The cosmological constant, representing the energy density of empty space, is vastly smaller than predicted by quantum field theory, requiring an unexplained cancellation of enormous contributions.
The exploration of alternative gravity theories is motivated by the desire to address these shortcomings and provide a more natural and complete explanation of the universe. MOND, for instance, offers a compelling alternative to dark matter at galactic scales. However, it has difficulties explaining the observed properties of galaxy clusters and the cosmic microwave background. Furthermore, MOND lacks a fully consistent relativistic formulation, making it challenging to test in strong gravitational fields.
Tensor-vector-scalar (TeVeS) gravity is one attempt to provide a relativistic extension of MOND. TeVeS introduces additional fields that interact with gravity, modifying its behavior at low accelerations. While TeVeS can reproduce some of MOND’s successes, it is more complex and faces challenges in explaining certain cosmological observations.
Other modified gravity theories, such as f(R) gravity, modify Einstein’s field equations by introducing a function of the Ricci scalar (R). These theories can potentially explain the accelerated expansion of the universe without invoking dark energy. However, they often suffer from instabilities and require careful tuning of parameters to match observations.
Braneworld models offer another intriguing approach to modifying gravity. These models propose that our universe is a four-dimensional brane embedded in a higher-dimensional space. Gravity can propagate into the extra dimensions, leading to modifications of its behavior at large distances. Braneworld models can potentially explain both dark matter and dark energy, but they also introduce new challenges related to the stability and consistency of the extra dimensions.
The search for a more complete theory of gravity is closely intertwined with the ongoing efforts to detect dark matter particles directly. Numerous experiments are underway to search for weakly interacting massive particles (WIMPs), axions, and other dark matter candidates. If dark matter is detected, it would provide strong support for the Lambda-CDM model. However, if dark matter remains elusive despite these efforts, it would further motivate the exploration of alternative gravity theories.
The distinction between modifying gravity and adding new particles to the universe isn’t always clear-cut. Some theories propose that dark matter interacts with gravity in unexpected ways, leading to modifications of its observed behavior. For instance, self-interacting dark matter can affect the distribution of dark matter in galaxies and clusters, potentially resolving some of the discrepancies between simulations and observations.
The ongoing debate about the nature of gravity highlights the importance of continued research and experimentation. New observations from telescopes and space-based missions are providing unprecedented insights into the structure and evolution of the universe. Theoretical physicists are developing new models and refining existing ones to explain these observations. The interplay between theory and experiment is essential for advancing our understanding of gravity and the universe.
The potential implications of rewriting gravity extend beyond cosmology and astrophysics. A deeper understanding of gravity could have profound implications for fundamental physics, potentially leading to new insights into the nature of spacetime, quantum gravity, and the unification of the fundamental forces. The quest to understand gravity is one of the most challenging and exciting frontiers in modern science.
Expanded Context and Background Information:
To fully appreciate the current re-evaluation of gravity, it’s crucial to understand the historical development of our understanding of this fundamental force. Isaac Newton’s law of universal gravitation, formulated in the 17th century, provided the first comprehensive description of gravity. Newton’s law explained the motion of planets around the Sun and the falling of objects on Earth with remarkable accuracy. However, it was based on the concept of instantaneous action at a distance, which was later challenged by Einstein’s theory of relativity.
Einstein’s general relativity, published in 1915, revolutionized our understanding of gravity by describing it as a geometric property of spacetime. According to general relativity, mass and energy warp spacetime, and objects move along the curved paths determined by this warping. General relativity made several groundbreaking predictions, including the bending of light around massive objects, the existence of gravitational waves, and the expansion of the universe. These predictions have been confirmed by numerous experiments and observations, solidifying general relativity as the most successful theory of gravity to date.
Despite its successes, general relativity faces several challenges. One of the most significant is its incompatibility with quantum mechanics. Quantum mechanics describes the behavior of matter and energy at the atomic and subatomic levels, while general relativity describes gravity at the macroscopic level. Attempts to unify these two theories into a single theory of quantum gravity have so far been unsuccessful.
The existence of singularities in general relativity, such as those at the center of black holes, also poses a challenge. At these singularities, the curvature of spacetime becomes infinite, and the laws of physics break down. This suggests that general relativity is incomplete and needs to be modified to account for these extreme conditions.
The mysteries of dark matter and dark energy represent another major challenge to general relativity. As mentioned earlier, these enigmatic entities constitute approximately 95% of the universe’s total mass-energy content, yet their nature remains unknown. The standard cosmological model, Lambda-CDM, relies on the existence of cold dark matter and a cosmological constant to explain the observed structure and expansion of the universe. However, the lack of direct detection of dark matter particles and the fine-tuning problem associated with the cosmological constant have led some physicists to question whether our understanding of gravity itself is flawed.
The alternative gravity theories mentioned earlier, such as MOND, f(R) gravity, and braneworld models, represent attempts to address these challenges and provide a more complete and consistent description of gravity. These theories modify general relativity in various ways, either by introducing new fields, modifying Einstein’s field equations, or postulating the existence of extra dimensions.
The search for a more complete theory of gravity is an ongoing process that involves both theoretical and experimental research. Theoretical physicists are developing new models and refining existing ones to explain the observed properties of the universe. Experimental physicists are conducting precise measurements of galaxy rotation curves, gravitational lensing, and the cosmic microwave background to test these models.
The potential implications of rewriting gravity are far-reaching. A deeper understanding of gravity could not only solve the mysteries of dark matter and dark energy but also revolutionize our understanding of cosmology, astrophysics, and fundamental physics. It could lead to new insights into the nature of spacetime, quantum gravity, and the unification of the fundamental forces.
Quotes from the Original Source (Attributed):
While the original Yahoo News article doesn’t contain direct quotes from named researchers, it does offer a succinct summary of the situation: “We might be completely wrong about gravity.” This statement encapsulates the central idea of the article, which is the growing recognition within the scientific community that our current understanding of gravity may be incomplete.
Experiments and Observational Evidence:
Numerous experiments and observational programs are underway to test the validity of general relativity and to search for evidence of modified gravity. These include:
- Galaxy Rotation Curves: Astronomers are measuring the rotation speeds of stars in galaxies to determine the distribution of mass. The observed flat rotation curves of galaxies, where stars orbit at roughly the same speed regardless of their distance from the galactic center, suggest the existence of dark matter or modified gravity.
- Gravitational Lensing: The bending of light around massive objects, known as gravitational lensing, can be used to map the distribution of dark matter and to test the predictions of general relativity.
- Cosmic Microwave Background (CMB): The CMB is the afterglow of the Big Bang and contains valuable information about the early universe. Precise measurements of the CMB can be used to constrain cosmological parameters and to test different gravity models.
- Gravitational Waves: The detection of gravitational waves by the LIGO and Virgo collaborations has opened a new window into the universe. Gravitational waves can be used to probe strong gravitational fields and to test the predictions of general relativity.
- Space-based Missions: Space-based missions, such as the European Space Agency’s Euclid telescope, are designed to map the distribution of galaxies and dark matter with unprecedented accuracy. These missions will provide a wealth of data to test different gravity models and to shed light on the nature of dark energy.
Frequently Asked Questions (FAQ):
Q1: Is Einstein’s theory of general relativity wrong?
No, it’s not necessarily “wrong,” but it may be incomplete. General relativity accurately describes gravity within our solar system and in many other situations. However, it struggles to explain phenomena like dark matter and dark energy without requiring the existence of these mysterious substances. Physicists are exploring modifications and alternatives to general relativity to address these shortcomings.
Q2: What are dark matter and dark energy, and why are they a problem for general relativity?
Dark matter and dark energy are hypothetical entities that make up about 95% of the universe’s mass-energy content. We infer their existence from their gravitational effects, but we haven’t directly detected them. Dark matter is thought to provide extra gravity to hold galaxies together, while dark energy is believed to be causing the universe’s accelerated expansion. These concepts pose a challenge for general relativity because they require the introduction of components whose fundamental nature is unknown, leading some to suspect that our understanding of gravity itself needs revision.
Q3: What is MOND, and how does it differ from general relativity?
MOND stands for Modified Newtonian Dynamics. It’s an alternative theory of gravity that proposes that at very low accelerations, gravity deviates from Newtonian predictions. This modification could explain the observed flat rotation curves of galaxies without invoking dark matter. MOND primarily affects galactic scales, while general relativity focuses on the behavior of gravity in stronger fields and over larger cosmic distances.
Q4: What kind of experiments are being done to test these alternative gravity theories?
Scientists are conducting various experiments and observations to test these theories. These include precise measurements of galaxy rotation curves, gravitational lensing, and the cosmic microwave background. Space-based missions like the Euclid telescope are mapping the distribution of galaxies and dark matter with unprecedented accuracy, providing a wealth of data to test different gravity models. The detection of gravitational waves also offers a new way to probe strong gravitational fields and test the predictions of general relativity and its alternatives.
Q5: If gravity is rewritten, what impact would that have on our understanding of the universe?
Rewriting gravity could have profound implications for our understanding of the universe. It could potentially solve the mysteries of dark matter and dark energy, provide a more complete and consistent description of cosmology, and revolutionize our understanding of fundamental physics. A new theory of gravity could also lead to new insights into the nature of spacetime, quantum gravity, and the unification of the fundamental forces.
Concluding Remarks:
The pursuit of a complete understanding of gravity is an ongoing scientific endeavor marked by both theoretical innovation and observational breakthroughs. While Einstein’s general relativity remains a cornerstone of modern physics, the persistent mysteries of dark matter and dark energy have spurred researchers to explore alternative models that challenge our conventional understanding of the universe’s fundamental forces. The search for a more comprehensive theory of gravity promises to reshape our understanding of the cosmos and the very fabric of spacetime. The implications of rewriting gravity are profound, potentially revolutionizing cosmology, astrophysics, and fundamental physics, ultimately leading to a deeper and more nuanced view of the universe.